How Body-on-a-Chip Tech Is Transforming Research With Professor Donald Ingber
Miniature models that grow on microchips could replace animal testing and replicate the complex functionality of real organs, such as the vagina.
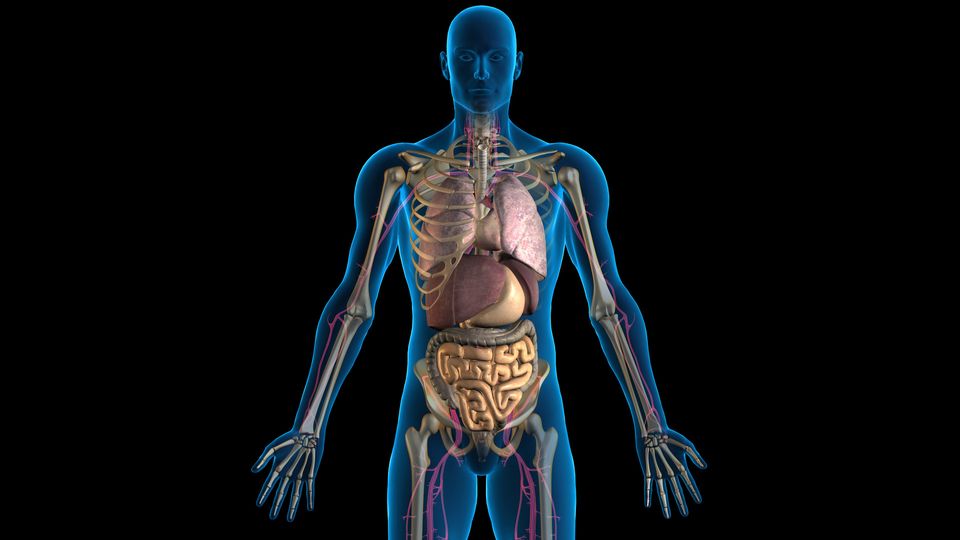
Complete the form below to unlock access to ALL audio articles.
Professor Donald Ingber, founding director of the Wyss Institute for Biologically Inspired Engineering at Harvard University, is responsible for the development of human organ chips. These organ chip systems provide researchers with a window into inter-organ physiology and the body’s response to drugs, without the need for animal testing.
These chips can be applied to drug development, disease modeling and personalized medicine.
Technology Networks invited Ingber to an Ask Me Anything session to answer your questions about this incredible technology.
Lucy Lawrence (LL): What is a body-on-a-chip system?
Donald Ingber (DI): So, we call them organs-on-chips or organ chips, and if you link them together, you can get a body-on-a-chip. The organ chips that we first developed were small devices the size of a computer memory stick. They are optically clear and made out of a flexible polymer silicone rubber. They have two hollow tiny channels less than a millimeter wide that run parallel on top of each other and are separated by a porous membrane. We can put different cell or tissue types on either side [of the membrane] to create tissue‒tissue interfaces, which is why we call it an organ-on-a-chip rather than a tissue-on-a-chip.
Figure 1: A three-layer microfluidic chip model with cells grown on a porous membrane. Credit: Technology Networks, adapted from Organs-on-Chips: Furthering Our Understanding of Disease.
We are able to stretch and relax the membrane and attached tissues laterally, so we can mimic breathing motions if we have air above. If we have fluids on both sides, we can mimic peristalsis or deformation in any tissue.
We've been able to mimic physiology and disease states, drug actions and toxin actions with incredible fidelity.
If you flow medium through the vascular channel line by endothelial cells, through the chip, you could take the effluent and put it into the inflow part of the next chip, to create a body-on-a-chip. This allows you to look at multi-organ coupling.
LL: What sparked your interest in body-on-a-chip technology and organ-on-a-chip technology?
DI: When I was an undergraduate student, I had insight that mechanical forces might be as important for cell shape as chemicals and genes, and that led me on a path to experimental testing. Eventually, I convinced people that it was true by developing ways to control cell shape via distortion or stretching. In our first paper published in 1994, we adapted computer microchip manufacturing techniques to gain control over features at the same nanometer/micrometer scale at which cells live. We also created a technique to control how far cells can spread by making islands coated with extracellular matrix the size of cells. That's how we got into microchips and cell biology. We then started making hollow channels that are called microfluidics and then we began culturing cells in those channels.
LL: What are the challenges or limitations that you've come across while developing and using your body- and organ-on-a-chip systems?
DI: With organ-on-a-chip, the biggest limitation we faced were bubbles. The tubes are very small, so, if you get bubbles in them by connecting and disconnecting tubes, this can cause the cells to die. In efforts to commercialize organ-on-chip systems, “click and play” approaches are being developed that don’t have connectors. This has made the technology much more robust, and has helped enormously.
Cell sourcing is always a challenge, whatever in vitro model you use, we find that organoids and primary adult cells have been the best over induced pluripotent stem cells because they retain epigenetic signatures and disease states.
Right now, the chips are relatively low throughput, but incredibly high content.
Whole body-on-chips have additional problems, as you now have to use multiple cell types for culturing and you have to time them so that they can be put on the chips, differentiated and all aligned so the chips can be coupled together.
But developing noninvasive readouts, whether we use electrodes, oxygen sensors or fluorescent reporters, are challenges that you would face in any culture system.
Another challenge is the amount of data generated, similar to an animal model, and for people used to 96-well plates with quick assays, it's very different.
LL: Can organ chips be used to study microbial infectious diseases?
DI: Absolutely. Not only can we study pathogens, but we can also study healthy microbiome versus diseased microbiome. Another major advantage of microfluidic organ chips as opposed to microphysiological systems is the ability to keep a complex microbiome in direct contact with live human cells for multiple days. If you took an organoid and put microbes on it, you would have about 16 to 24 hours before they die.
So, we've used diluted stool samples from patients in the lumen of our intestine chips. If the microbiome is healthy, they remain intact for five days. If it’s unhealthy, you will see blunting of the villi, compromised barriers and inflammatory cytokines.
We recently published a paper on a vagina chip with healthy microbiome versus disease, and we're using the chip with the Gates Foundation to test live biotherapeutic products.
These products for bacterial vaginosis are moving to clinical trials in South Africa and the United States in the next few months, and we can see these actually suppress inflammation on these chips.
In terms of pathogens, we've done viral infection and bacterial infection of intestine chips. We use metabolomics and can figure out which metabolites mediate the microbiome. In this case, we were looking at human microbiome protecting against enterohemorrhagic E. coli versus mouse microbiome, because humans and mice have totally different sensitivity.
LL: Could your replica models be linked to an artificial intelligence (AI) environment for studying and testing?
DI: We have papers in preprint and in development where we used machine learning and AI approaches. In these studies, we started with transcriptomic data from patients to see which genes across the whole transcriptome would need to be “flipped” to make faulty genes healthy. We then looked through all the available transcriptomic data on the web for every known drug, and then the AI machine learning computational tool prioritizes which [drugs] are most likely to reverse the state. These can then be explored more using in vitro models, including organ chips, to get transcriptomic data on human models.
The interesting thing about the computer tool is that it also analyzes known gene networks and known gene drug networks, and you can identify drug targets and then not only repurpose existing drugs, which we've done and moved to clinical trials, but actually identify new targets and identify new drugs.
We also do it the other way around, by which we use a molecular dynamics simulation for drug molecular design, and then we integrate machine learning and AI, in this process. We use organ chips coupled with it to generate human data.
Professor Don Ingber was speaking to Lucy Lawrence, Senior Digital Content Producer for Technology Networks.